(Click
on underlined link
to go to subject)
-
Contents
Chapter
1. Vision System Design
Chapter
2. Biological Eye Designs
A.
Camera
B.
Pinhole
C.
Concave
mirror
D.
Apposition
E.
Neural
superposition
F.
Refraction superposition
G.
Reflection superposition
H.
Parabolic superposition
I.
Multiple
sensor types
and combinations of types
Chapter
3. Eye Design Illustrations
Chapter
4. Eye
Reproduction
Chapter
5. Optical
Systems
Design
Chapter
6. The Eye Designer
Related Links
Appendix
A - Slide Show & Conference Speech by Curt Deckert
Appendix
B - Conference Speech by Curt Deckert
Appendix
C - Comments From Our Readers
Appendix
D - Panicked Evolutionists: The Stephen Meyer Controversy
|
EYE
DESIGN BOOK
Chapter 2
Sections D, E and F
-
Prev Page
Go
to Chapter
Links
-
Next Page
-
(Click on PICTURE
IN TEXT
to bring up LARGE PICTURE)
2. BIOLOGICAL EYE
DESIGN
D. Apposition compound
The apposition
compound eye design does not form a complete image in each facet. Each
facet picks up part of the image. It is compound because there are many
facets or separate sensor modules. It is one of the more common small
eye designs. It occurs in insects such as ants, wasps, dragonflies,
bees, and cockroaches. Each of many small facets or lenses of the eye
are separate light sensors. The brain puts each image
together by processing the output of each sensor of each
small lens facet. Each facet lens contains its own sensors to detect
light from specific angles. For small eyes, this is an extremely
complex approach. Each very small eye sensor facet or module has a lens
and light pipe to gather each part of the total image. Signals from
each facet of the image are then relayed to the brain.
One advantage of this type of
compound eye is the ability to detect movement within a partial scene
while using less brain processing time than that required to process
the full image. An advantage over camera and pinhole eyes is that they
can cover very wide fields with less total volume, because of the
shortening of the optical path of each facet. Parts of these eyes are
similar to lens arrays and new camera sensors now being developed for
small advanced cameras, but the integration of the apposition design is
difficult. Figure 2.9 helps us to visualize how these eyes are
designed. (P. 359, Physiology
of Photoreceptor Organs, 1972,
Ed. by M. G. F. Fuorki, Pub. by Springer-Verlag)
(Figure 2.9a: For reference see Fig 7.3, p. 128, Animal
Eyes, Michael F. Land, Dan-Eric
Nilsson, Oxford Animal Biology series, Oxford University Press, 2002) |
Figure
2.9 Apposition
Compound Eye Design.
Figure
2.9a Apposition
Eye Construction.
|
Fig
2.9b Diffraction,
Resolution, & Eye Size
(Reference: Figure 7.6, p. 134, Animal
Eyes, Michael F. Land, Dan-Eric
Nilsson)
|
Figure
2.9c Equivalent
Compound Eye
Applied
To Human Resolution. (Reference:
Figure 7.7, p. 136, Animal Eyes,
Michael F. Land, Dan-Eric Nilsson)
|
Figure
2.9e Light Adaptation
Of Compound Eye.
(Reference: Figure 7.9, p. 139, Animal
Eyes, Michael F. Land, Dan-Eric
Nilsson, Oxford Animal Biology series, Oxford University Press, 2002-
Please see their book for more details )
|
An example of
limitations on each element of a compound eye is shown by the above Figure
2.9b Diffraction, Resolution, & Eye Size
If we take the
technology of a compound eye, appearing on many insects, and applied it
to the requirement for human eyesight we would get an eye that is
extremely large. Then if we allow the resolution to fall off with angle
the eye is still so large that it would dominate the human head.This is
shown on the above Figure 2.9c
Equivalent Compound Eye Applied To Human Resolution
Compound eyes have
unique ways of adapting to changing light. Many of these are quite
diverse and indicate very sophisticated design at the micron levels.
The above Figure 2.9e Light
Adaptation Of Compound Eye
illustrates several ways that compound eyes adapt to light.
Some crabs use this design
along with some other
optical designs such as distributed eyes. Compound apposition eyes of
insects
and sea animals contain sensors within each facet. Each sensor contains
a crystalline lens and a small gradient index light pipe. The following
are examples of several creatures having compound eyes. The facets
making
up each creature's eyes are shown for comparison with respect to the
role
of each in creating a complete image.
Creature |
Number of
Eye Facets |
Role Requirements for Vision |
wood lice
small flies
lobster
dragonfly |
25
5,000
14,000
30,000 |
simple 2-dimension movement
complex
3-dimension movement
complex
2-dimension movement
complex
3-dimension movement |
Since the lens and light pipe of
each facet is very small, light may form interference patterns in these
narrow light guides. Some eyes even take advantage of wave patterns
within
facets to get more efficiency. This means that small eye facets have to
be reproduced with very close tolerances for clear vision to occur. An
illustration of a dragonfly having apposition compound eyes is shown in
Figure 2.10. (P. 17 left top, Megabugs,
The Natural History Museum book
of insects, Barnes &
Noble, N.Y., Miranda MacQuitty with Laurence
Mound) |
Figure
2.10. Example of
Apposition Compound
Eyes
|
E.
Apposition-Neural Superposition compound
The neural or brain
superposition eye has
a number of small lenses arranged in a compact pattern. Each lens
collects
light from only a small part of the total field of view. Using the
neural
superposition approach, the brain puts all the small images together
from
the individual sensor outputs of a large number of small eye facets.
Each
facet contains very small light guides and sensors. Each of these
facets
or sets of sensors has its own optics and unique angular
optical
axis to pick up part of a scene, but multiple facet light inputs are
combined
for each segment of the image. Each segment or pixel of a scene is
visualized
by a series of different sensors contained in multiple facets. Output
signals
from combined facets can provide more sensitivity for better vision at
low light levels. Typically, small flies will have this type of optical
design. Where size is critical and wide-angle fields of view are
required,
this design is more suitable than camera eyes for small low-resolution
applications. Figure 2.11 illustrates the slightly different optical
design
of the neural superposition eye. Image processing would need to be
unique
for this type of eye. Image processing is complex for any eye, but here
it is even more amazing when you consider the small brain size of flies
having this type of optical design. (p. 303, Fig. 3b,
Vision Optics
& Evolution by Dan E.
Nilsson, Biosciences, Vol. 39, No. 5, May
1989)
This optical design requires
considerable
parallel processing of visual data to provide image recognition with
quick
response. These eyes can operate at lower light levels when they can
use
the combined output from multiple facets for each point of an image to
achieve greater sensitivity. (An example of an application of the
neural
superposition eye is shown in Figure 2.12 taken from Fig. 44, P.495,
Chapter
8 PhotoChemistry of Vision,
Ed. by Herbert J. A. Dastnall, Springer-Verlag,
N.Y. 1972. Figure 3.12b courtesy of www.pbrc.hawaii.edu/bemf
(c)MicroAngela )
Figure
2.11 Neural
Superposition Eye.
(Like Fig. 6-5)
|
Figure
2.12a Apposition
Neural
Superposition
Simplified Small
Fly Eye
|
Figure
2.12b Compund
Insect Eye
|
F. Refraction
superposition compound
Like the previous design, this
optical design would also be difficult to adapt from other eye designs.
These eyes occur in moths, some flies, many beetles, and some shrimp.
This optical design may be a more difficult eye to integrate, but it
does give considerable design flexibility for small insect vision. It
is a design where an array of clear refractive (or transmitting)
crystalline cones work together as telescopic optics to produce a
reversed image on a very small retina.
Figure
2.13 Refraction
Superposition Eye
|
Figure
2.13a Reflective
Superposition
(See Figure 8.14, p. 173, Animal
Eyes.)
|
Figure
2.13b Reflective & Refractive Superposition (See
Figure 8.14, p. 173, Animal
Eyes.)
|
(Figure 2.13
illustrates the refraction superposition optics techniques is from p.
303, Fig. 3c, Vision Optics
& Evolution by Dan E.
Nilsson, Biosciences, Vol. 39, No. 5, May 1989. For more details on figures
2.13a and 2.13b Refer to Animal
Eyes, Michael F. Land, Dan-Eric
Nilsson, Oxford Animal Biology
series, Oxford University Press, 2002)
The intricate detail of
reflective superposition eyes is shown on the above Figure
2.13a Reflective Superposition Example.
Current MEMS technology can allow some fabrication of similar
structures and optics, but the processing features indicate software
intelligence that is very significant for the size of the brain
interpreting the input from the eyes.
Two different approaches to
Superposition Eyes are shown in the above Figure
2.13b Reflective And Refractive Superposition.
The refraction superposition
compound optical design is similar to gradient index lens arrays, which
are currently used to replace conventional lenses where optical
distances are very short and where broad field coverage is needed.
Gradient index lenses can greatly reduce the optical path of an optical
system where an upright image is required. Like very small arrays of
lenses, gradient index material allows imaging over short distances.
Here facets may not require precise spherical surfaces. Images are
corrected by light being bent by very repeatable radial variations in
the refractive index of the light guide material within each facet.
These arrays of gradient index facets refract light much like an array
of small lenses.
Gradient index material occurs
in many natural eyes. Control of repeatable radial material variations
at the cell level is required to make functional imaging systems. There
is very little probability of gradient index cell arrangements
occurring randomly in eye facets without considerable intelligent
control. Each facet forms the equivalent of a small Kepler telescope
that contains the equivalent of two lenses to make the image upright.
Figure 2.14 shows several examples of refracted super position. (Fig.2.14
is from P. 97 left top, Megabugs,
The Natural History Museum book of insects,
Barnes & Noble, N.Y., Miranda MacQuitty with Laurence Mound. Fig.
2.15a is from Fig. 12, P.459,
Chapter 8 PhotoChemistry of
Vision, Ed. by Herbert J. A.
Dastnall, Springer-Verlag, N.Y. 1972)
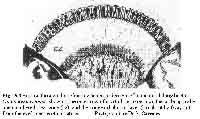
Figure
2.15b Representative Superposition Eye Section
(For details see Figure 8.1, p. 156, Animal
Eyes,
|
Figure
2.15c Superposition Eye Dark Adaptation Methodology
(For details see Figure 8.9, p. 165, Animal
Eyes).
|
|
Figure
2.14 Refracted
Superposition
Moth
Eye with a large
number of
facets (Like Fig.
3-21)
Figure
2.15a Detail of Moth
Eye Facets
|
The above Figure
2.15b shows a section of a
superposition eye. It indicates very complex approach that some optical
designers have tried to emulate. There are many applications for
optical systems of this type, if they could be replicated economically.
Such an optical system can be built in a very small volume. This type
of eye allows small insects and some larger animals to have a wide
field of view.
The above Figure 2.15c
illustrates how the superposition eye adapts to various levels of
light.
(Reference: Animal Eyes,
Michael F. Land, Dan-Eric Nilsson, Oxford Animal Biology series, Oxford
University Press, 2002- Please see their book for more details )
Questions
for Discussion
Do you wonder, like
I do, what has controlled the design of eyes |
|